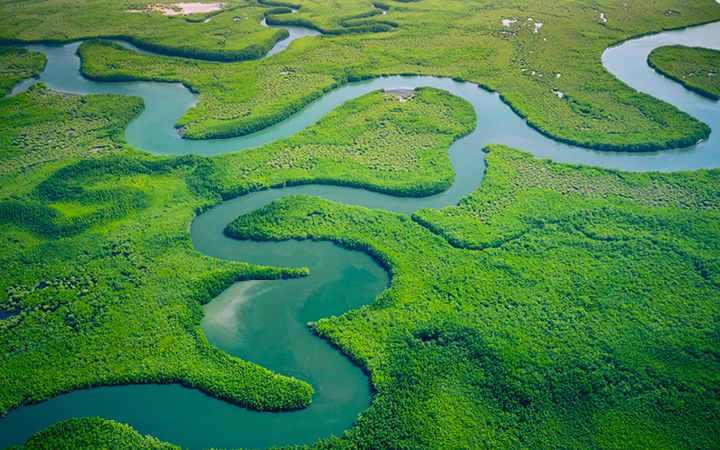
Exploring hydrogen as a versatile option for decarbonization
Given the increasing focus on decarbonization, stakeholders across the energy industry see potential for hydrogen. As they look to the future of energy, many are asking: What is the hydrogen value proposition? Where does it best compete? What will be the key technological challenges?
Simply put, the value proposition for hydrogen is in its versatility. While there are technical challenges and other obstacles for hydrogen to realize its full potential in the energy industry, there are also many opportunities for hydrogen to play an important role in a low- to zero-carbon energy transition.
To understand the hydrogen potential, it’s important to first break down its three lifecycle phases: production, infrastructure and transport, and end use.
- Production: Currently, production comes from a process known as steam methane reforming (SMR), with hydrogen and carbon dioxide as the principal products. Other production approaches, like electrolysis and thermochemical water splitting, account for less than 10% of total U.S. hydrogen production.
- Transportation: With 1,600 miles of dedicated hydrogen pipelines in the United States, pipelines are the main method for transportation. Other transport opportunities include truck or rail for specialty applications.
- End uses: Presently, this primarily includes petroleum refining, agriculture, manufacturing, and food processing. Across the energy industry, other potential end uses are being explored, from transportation to power generation.
Keeping the lifecycle categorization in mind, this article explores some of the near-term (within the next five years), medium-term (5-10 years), and longer-term (more than 10 years) opportunities for hydrogen throughout the energy lifecycle.
Demand and production
Hydrogen quick reference
- Current U.S. demand is more than 10 million metric tons annually.
- On a mass basis, hydrogen has nearly three times the energy content of gasoline. Meaning, a kilogram (kg) of hydrogen contains 33 kWh of usable energy, whereas gasoline and diesel only hold about 12 kWh/kg.
- At normal temperature and pressure (20º C and 1atm), 1 kg of hydrogen has a volume of 11m3, while 1 kg of gasoline has a volume of 0.0013 m3. Hence the need to compress or liquify hydrogen for storage and transportation.
The status quo
The primary method for producing hydrogen today is a process known as steam methane reforming (SMR). Through SMR, natural gas is leveraged as methane to produce hydrogen and carbon dioxide as the principal products. Recently, the inclusion of carbon capture and storage (CCS) technology following the SMR process drew attention as a way to reduce the overall carbon dioxide by-production. CCS technology has the potential to capture approximately 95% of the carbon dioxide generated during the hydrogen production process.
- The current cost of SMR hydrogen is ~$0.70/kg H2 ($6.20/MMBtu). Hydrogen produced through SMR with CCS technology is anticipated to achieve of cost of ~$1.35/kg H2 ($12/MMBtu) or less by 2025.
- Within the decade, hydrogen produced with zero or negatively priced electricity and electrolyzers is anticipated to achieve a cost of $1.20/kg H2 ($10.60/MMBtu) or less by 2030.
- Hydrogen produced with renewable electricity and electrolyzers is anticipated to achieve a cost of $2/kg H2 ($17.70/MMBtu) or less by 2030.
People often call SMR with CCS “blue” hydrogen, generally considered a clean hydrogen supply solution. Researchers continue to evaluate the net environmental impacts of some hydrogen production pathways—like blue hydrogen—as these technologies develop. Presently, the bipartisan infrastructure bill under consideration by the U.S. Congress defines low-carbon hydrogen as that which emits less than 2.5 kg of CO2 per 1 kg of H2 produced.
Tailwinds for alternate hydrogen production options
The precipitous drop in the cost to generate electricity from wind and solar increases the viability of electrolysis as an option to produce zero-emissions hydrogen. In this scenario, electricity and water are the electrolysis inputs, with hydrogen and oxygen as the resulting output. In addition to declining renewable electricity costs, the cost of electrolyzers also decreases with the rise of global manufacturing capacity. If the electricity comes from renewable sources such as hydro, wind, or solar, the result is commonly referred to as “green” hydrogen since there are no carbon emissions generated.
Promising production potential
There is significant industry discussion around green hydrogen. But, there’s also a near-term business case for using excess electricity that otherwise might be curtailed—from renewable energy projects or otherwise—to run electrolyzers that generate hydrogen. Such a scenario leads to hydrogen production from electrolysis that could be competitive with SMR hydrogen before 2030.
Similarly, there are financially viable, near-term scenarios in which excess capacity from nuclear plants powers electrolyzers, creating what is commonly referred to as “pink” or “yellow” hydrogen. This business opportunity continues to be an area of focus, especially for existing nuclear asset owners.
If demand for hydrogen grows, we expect that gasification or pyrolysis (gasification in the absence of oxygen) hydrogen production processes will emerge over the next 10 years. Demand for hydrogen is required to help facilitate the evolution and development of gasification technology. Such hydrogen production processes can use biomass—which consumes carbon dioxide while it grows—or municipal waste as feedstocks. This is commonly referred to as “turquoise” hydrogen. As gasification technology evolves, the investment to pilot and commercialize new advancements increases. There is longer-term potential for other production methods, such as high-temperature thermochemical water splitting.
A colorblind continuum
Hydrogen production mechanisms stand to compete against one another as the hydrogen ecosystem evolves. Along the way, hydrogen must also compete with other fuels, including natural gas and diesel. While the color terminology assigned to hydrogen helps identify production technologies, by necessity, the energy industry needs to be colorblind. The color focus distracts from the fact that hydrogen as a fuel has the same functionality regardless of production approach, with a range of carbon intensities based on the particulars of its production process.
Rather, the industry needs an increased focus on metrics and methodology to quantify carbon emissions so that stakeholders can assess the value of low-cost energy options along with carbon emissions. These assessments are critical to developing a system that can track hydrogen molecules from the source or production to consumption to help with such valuation. Policy and regulations designed to support the production and valuation of hydrogen production methods with greater carbon reduction or even carbon-negative potential is also an area that requires increased focus.
As part of the policy and finance equation, the value and revenue streams associated with hydrogen production will expand. For example, if a national carbon policy is established, carbon capture paired with hydrogen production could provide an additional revenue stream. Similarly, oxygen streams could be another source of potential revenue as it is produced alongside hydrogen by electrolyzers. Oxygen streams use potential includes oxy-firing combustion engines, gasification or biofuel processes, or industrial applications.
Infrastructure
A key constraint for expanding the integration of hydrogen into the energy economy is its transportation and the infrastructure needed to move it from production locations to places where it is in demand as end use categories expand beyond historical hydrogen demands.
Proponents of the hydrogen industry point to electricity transmission studies from the late 1990s and 2000s as an analogy. At that time, the standard belief was that a maximum of 15%-20% electricity from renewable sources could be integrated into the electricity grid before creating widespread negative impact. But as of 2020, approximately 20% of electricity generated in the U.S. came from renewable sources. Several European nations achieved penetration of up to 50% renewable electricity, with clear roadmaps and implementation plans to push those levels even higher.
While some commercial and technical challenges exist, those challenges are addressed by mitigants. This represents the learning curve that comes with increasing amounts of intermittent generation integrated into the electrical grid. Similarly, academia and national labs are already studying the potential for increased integration of hydrogen and the repurposing of the extensive existing infrastructure.
Proposition for pipelines
The U.S. has 1,600 miles of dedicated hydrogen pipelines for the transport of pure hydrogen, primarily for petroleum refining processes. There are also limited networks for the transportation of gaseous or liquid hydrogen by truck or rail.
Leveraging the approximately 2.5 million miles of existing natural gas pipelines that crisscross the U.S. represents one of the clearest ways to integrate hydrogen into our existing energy systems.
As one example, Hawaii Gas’ existing pipeline network accommodates up to 15% hydrogen. The benefit to blending low-carbon hydrogen with natural gas is a reduction of the overall carbon emissions at the end-use point. Pipeline transportation of hydrogen will ultimately be the most cost-effective way to transport hydrogen in the long term, either as a blended product or as pure hydrogen. However, to achieve that transportation method significant investment in and repurposing of existing infrastructure will be required.
Other hydrogen transportation options
Along with trucking hydrogen gas or liquid, another means of hydrogen transportation and storage is liquid organic hydrogen carriers (LOHC). LOHC is a liquid carrier with properties similar to diesel or gasoline. In this scenario, hydrogen chemically binds through a hydrogenation process and releases through a dehydrogenation process. Both processes require a catalyst. LOHC can absorb, hold, and release hydrogen, and is reusable over many hundreds of cycles for the transport and storage of hydrogen. Such technology allows for the transportation of hydrogen at ambient temperatures and pressures. Over the next decade, LOHC will play an increased role in the transportation and storage of hydrogen.
Solutions for long-duration storage
Hydrogen is also under consideration as a contender for long-duration storage to support the reliability and resiliency of both the electricity and fuel supply networks. Hydrogen is often difficult to store and must either be compressed or liquefied. But, if stored in a pure state, it is a generally accepted principle of physics that molecules are easier and cheaper to store than electrons.
Molecules are also more easily stored for long durations and in quantities dispatched over long durations: storage can take place for weeks to a month and dispatch can last for more than 12 hours. Hydrogen storage can include tanks or vessels, salt caverns, or even repurposed depleted natural gas wells. Hydrogen can also convert to ammonia (NH3), which is an easier molecule to store and transport. LOHC has also gained significant attention as a potential storage mechanism for hydrogen.
Overall, options for the transportation and storage of hydrogen are driven by regulation, transportation efficiency, and storage. Infrastructure investments will need evaluation with additional complexity, with consideration to leveraging or repurposing existing assets and infrastructure rather than building new and dedicated systems. One example is hydrogen storage and uses in combustion turbines—supporting electricity grid reliability in addition to battery storage applications. We anticipate discussion and decisions between electrical infrastructure versus hydrogen infrastructure.
End uses
End-use sectors for hydrogen in the near-term energy economy fall into four categories:
- Current uses include fuels refining and/or production, fertilizer production, industrial processes (steel, concrete, food processing), etc.
- Commercial and residential applications such as heating or backup power
- Transportation includes medium and heavy-duty vehicles and aviation
- Synthetic fuels for stationary power and transportation applications
One of the most viable business cases for hydrogen at present is the ability to leverage it to reduce carbon emissions in applications where electrification is not feasible. Renewable energy solutions through existing commercialized technologies can take us down the path to achieving stated climate goals. But, in industrial and transportation sections, achieving decarbonization will require solutions outside of system electrification.
Today’s hydrogen consumers: Industrial and manufacturing
Hydrogen supplies are presently limited, with most production reliant on demand for conventional applications.
Today, the primary end uses for hydrogen include petroleum refining, agriculture, manufacturing and metallurgy, and food processing. Interest in low- or no-carbon hydrogen, for which the carbon footprint of processes and products can be improved upon by displacing the current hydrogen supply from SMR, is gaining significant interest. For example, hydrogen is required for the production of steel. HYBRIT Development is a joint venture between SSAB, LKAB, and Vattenfall which seeks to develop fossil-fuel-free steel. It delivered “fossil-free” steel to Volvo to test in vehicle manufacturing. Further, a similar partnership with Mercedes-Benz plans to introduce fossil-free steel into vehicle production.
Commercial and residential applications
Blending hydrogen with natural gas or using pure hydrogen for end-use consumer and industrial gas-based equipment is more challenging than blending hydrogen in the midstream sector. This is a result of the compatibility of existing burners with the differing gas properties of hydrogen, including energy density and flame speed. The market is growing, as demonstrated in projects like the U.K.’s Hy4Heat program and Southern California Gas Co.’s H2 Hydrogen Home, The transition of commercial and residential appliances with high concentrations or pure hydrogen has potential, but it is on a longer-term trajectory than other hydrogen applications.
Comparatively, hydrogen use applications include combined heat and power (CHP) systems for industrial or commercial campus applications, or as backup power with fuel cells. This represents a near- to medium-term end use scenario. For example, 2G Energy installed its first CHP system to run on hydrogen at the Berlin Airport in Germany in 2014. Separately, Microsoft is exploring the use of hydrogen fuel cells for backup power at data centers, as well as synergistic use cases. This includes using electrolyzers to produce on-site hydrogen for grid services to the region utility or hydrogen sales at fueling stations for long-haul transportation. In the near term, decreasing costs of hydrogen production, as well as CHP systems and fuel cells, will be key drivers in the adoption of hydrogen for commercial and residential applications.
Transportation and synthetic fuels
For light-duty and passenger vehicles, battery electric vehicles (BEV or EV) and the associated infrastructure garnered significant attention over the last decade. The hydrogen equivalent is a fuel cell electric vehicle (FCEV) that stores hydrogen fuel in a tank to run a fuel cell, which generates electricity to power the vehicle. FCEVs are gaining attention for medium- and heavy-duty vehicles for delivery, municipal, or long-haul transportation.
While FCEVs gain recognition as competition to vehicles with internal combustion engines (ICE) or even BEVs, FCEV fueling infrastructure in the U.S. and globally is limited. In the nearer term, there is potential to incorporate hydrogen with existing diesel vehicles in a dual-fuel engine application. Low-speed, low-torque engine performance—found in delivery vehicles and municipal transport—allows hydrogen to burn, producing limited nitrous oxide (NOx). Existing vehicles can be retrofitted and existing diesel fueling infrastructure can continue to be leveraged, all while the integration of hydrogen into vehicles can have near-term impacts on reducing carbon emissions. ULEMCo is developing such retrofit technology for commercial vehicles.
Outside of ground transportation, there are also growing examples of hydrogen powering rail, air, and ocean transportation either through fuel cells or hydrogen as an input to produce low- or no-carbon synthetic fuels.
The value proposition
Hydrogen has several near-term and beneficial options for playing a role in decarbonization and energy transition. Ultimately, market conditions, technology development, and policy structures determine the extent of which production methods, infrastructure investments, and end uses are most viable. As stakeholders look for opportunities for decarbonization, hydrogen provides a versatile and significant opportunity for industries and consumers shifting toward a low- or zero-carbon energy solution.